By Luisa Cristini, PhD, University of Hawaii at Manoa
[Note from the editor: This is the second in a series of blog entries that will focus on introductory topics in climate dynamics and modeling, and will be a great insight into the current understanding of the science.]
Seventy-one percent of the Earth’s surface is covered by ocean, with an average depth of 3,700 m (12,139 ft). Three and a half percent of seawater is composed of dissolved salts, particles, gases, and organic matter. Chloride and sodium make up 85% of this dissolved material. To quantify the total amount of dissolved salts, the salinity is measured by the conductivity of seawater and given in practical salinity units (psu).
The density of seawater increases with increasing salinity and pressure and therefore with depth, while it decreases with increasing temperature. The temperature controls the changes in density at high temperatures, while the role of salinity is larger at low temperature. The salinity also influences the freezing point of seawater, which at the surface is 0°C (32°F) for pure water and -1.8°C (28.76°F) for saltwater at a salinity of 35 psu.
The surface ocean circulation is mostly driven by the wind. At mid-latitudes the atmospheric westerlies (winds blowing from the west) induce eastward currents in the ocean, while the trade winds (blowing in the Tropics from the northeast in the Northern Hemisphere and from the southeast in the Southern Hemisphere) are responsible for westward tropical oceanic currents. Because of the presence of the continents, the oceanic currents form quasi-circular loops called subtropical gyres, the most famous being the Gulf Stream off the eastern coast of the United States and the Kuroshio off the eastern coast of Japan. In the Southern Ocean (the waters surrounding Antarctica), because of the absence of continental barriers, the Antarctic Circumpolar Current (ACC) connects all ocean basins and is the strongest current on Earth, transporting one million cubic meters of water per second.
At high latitudes surface water has relatively high salinity and low temperature and thus can be dense enough to sink to great depths. This important process, called deep oceanic convection, is possible only in a few places around the world, mostly in the North Atlantic and in the Southern Ocean. In the former, North Atlantic Deep Water (aka NADW) is generated in the Labrador and Greenland-Norwegian Seas and flows along the ocean bottom southward along the western boundary of the Atlantic towards the Southern Ocean. From here it is then transported to other ocean basins by the ACC after some mixing and slowly upwells (rises) towards the surface. In the Southern Ocean, Antarctic Bottom Water (aka AABW) is produced in the Weddell and Ross Seas of Antarctica. This water mass is colder and denser than the NADW and therefore flows even further below it. Because of the mixing between the different water masses with different origins in the Southern Ocean, the water that finally enters the Pacific and Indian Oceans is normally called Circumpolar Deep Water (aka CDW).
This large-scale oceanic circulation associated with currents at all depths is named the thermohaline circulation, because it is driven by temperature (thermo-) and salinity (-haline) contrasts, with winds playing a secondary role at the surface. The thermohaline circulation is quite slow – it takes about a century for masses of water from the North Atlantic to reach the Southern Ocean, and often millenia to flow around the entire globe. The thermohaline circulation is the engine of Earth’s climate.
Because of the strong interaction between ocean and atmosphere, the sea surface temperature (SST) is close to the air temperature above it. One exception to this is in polar regions, where sea ice, formed from the freezing of the surface seawater, insulates the ocean from the colder atmosphere.
The sea surface salinity (SSS) is strongly influenced by freshwater fluxes. It reaches a maximum in the subtropical regions because of the low rainfall coupled with large evaporation (leaving salt behind). Higher precipitation induces lower salinity at the Equator, while weak evaporation leads to lower salinity at mid and high latitudes.
In winter, the stirring by the winds and the cooling at the surface both induce strong mixing in the ocean, homogenizing a surface layer called the oceanic mixed layer with fairly uniform water properties (temperature, salinity, conductivity, etc.) in depths up to 100 m (328 ft). In spring and summer, when temperatures rise and winds become weaker, the density at the surface decreases, thereby stabilizing the water column and causing the mixed layer to become shallower.
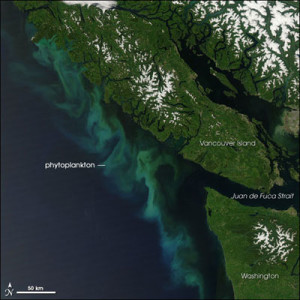
A phytoplankton bloom off the coast of the Pacific Northwest of North America as seen by satellite. Image reproduced from the Enyclopedia of Science.
The mixed layer dynamics, and in particular the seasonal changes of its depth, have great influence on the properties of surface water and on the exchanges of heat, water and gases between the atmosphere and ocean. Its development also has a large impact on the growth of phytoplankton (a type of organism that produces complex organic compounds from single inorganic molecules), which is the basis of the whole oceanic food web. Phytoplankton growth reaches its maximum during spring blooms, when the mixed layer is relatively shallow but the nutrient concentration is high thanks to the mixing with deeper layers that occurred during the previous winter.
Below the oceanic mixing layer, strong vertical temperature gradients are observed, showing that the majority of the ocean is strongly stratified. This means that light water sits above denser water and the water column is stable. In the deep ocean, the vertical gradients are much weaker. At the Equator, the temperature difference between the surface and a depth of 1,000 m (3,280 ft) could be more than 20°C (68°F), while the temperature difference between 1000 m and the ocean bottom is only on the order of 3°C (37°F)!
The temperature and salinity of seawater are modified by interactions with the atmosphere only in the oceanic mixing layer, and thus water mass modification and transformation occurs mainly at the surface. Water masses flowing beneath the mixing layer tend to keep their original properties. Consequently, the path of important water masses such as the NADW and the AABW can be followed from the region of their formation throughout the world’s oceans.
Reference:
Goosse H., P.Y. Barriat, W. Lefebvre, M.F. Loutre and V. Zunz, (2012). Introduction to climate dynamics and climate modeling. Online textbook available at http://www.climate.be/textbook.
Comments are closed.